
The University of Arizona and the European Southern Observatory, for example, have demonstrated that 4-meter-class mirrors, equipped with multiple actuators, can achieve wavefront errors of better than 53 nm rms (admittedly with substantially more rigid mirrors than those discussed in this report). The feasibility of using wavefront sensors and active-optics systems to control the figure of mirror surfaces has been proven by many groups. New techniques for figuring and testing allow rapid and inexpensive polishing of large mirrors to accuracies rivaling or exceeding the HST’s.

These new technologies allow us to make realistic plans for the next-generation of space telescopes -spacecraft with optical systems that are larger and lighter, yet much cheaper, than the HST’s. In the 15 years since the HST was built, enormous advances have been realized in the design, manufacturing, and testing of optical systems. The Hubble Space Telescope (HST) has vindicated many of these claims, but only at a very high cost. Images of astronomical bodies are not distorted by passage through Earth’s turbulent atmosphere, and, in principle, observations are not limited by vagaries of the weather or the diurnal cycle.
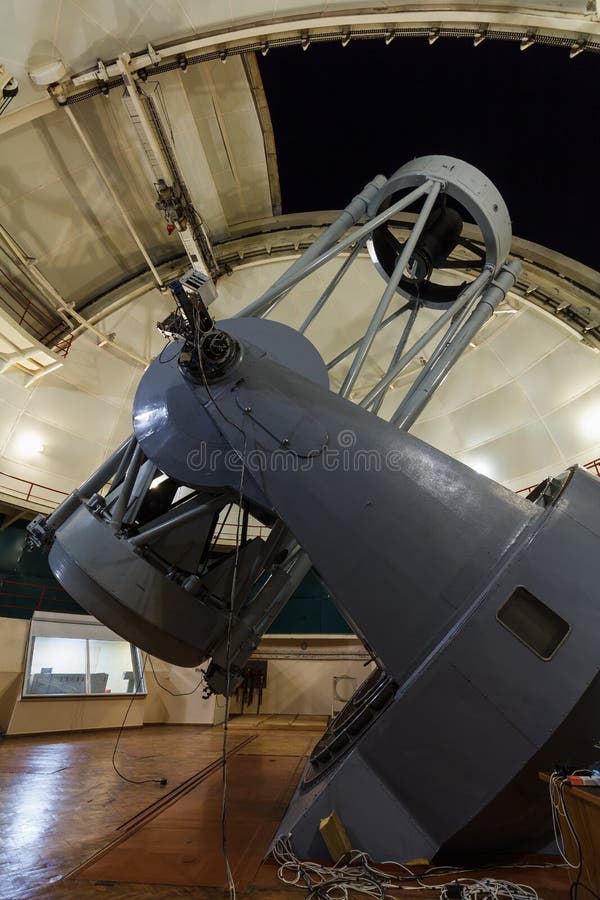
#Optical telescope 100nm full
From this vantage point it is possible to view the full span of the electromagnetic spectrum. Calculate the areas under each curve you've plotted.For decades astronomers have known about the advantages of locating astronomical telescopes in space. Try integrating Planck's equation at the top of the resource to give the area under any such curve. Using a simple graphics routine calculate representative spectra for each wavelength, temperature pair. The first equation at top for the problem set allows the calculation of spectral radiance. Pick representative wavelengths from each band or sub-band and calculate a matching temperature to complete the pair. Radio waves have frequencies from 300 GHz to as low as 3 kHz, and corresponding wavelengths from 1 millimeter to 100 kilometers. In all cases, microwave includes the entire SHF band (3 to 30 GHz, or 10 to 1 cm) at minimum, with RF engineering often putting the lower boundary at 1 GHz (30 cm), and the upper around 100 GHz (3 mm). This broad definition includes both UHF and EHF ( millimeter waves), and various sources use different boundaries. Microwaves, a subset of radio waves, have wavelengths ranging from as long as one meter to as short as one millimeter, or equivalently, with frequencies between 300 MHz (0.3 GHz) and 300 GHz. In wavelengths, this range corresponds to 0.1 mm (or 100 µm) infrared to 1.0 mm microwave.

The term typically applies to electromagnetic radiation with frequencies between high-frequency edge of the microwave band, 300 gigahertz (3 x 10 11 Hz)," and the long-wavelength edge of far-infrared light, 3000 GHz (3 x 10 12 Hz or 3 THz).
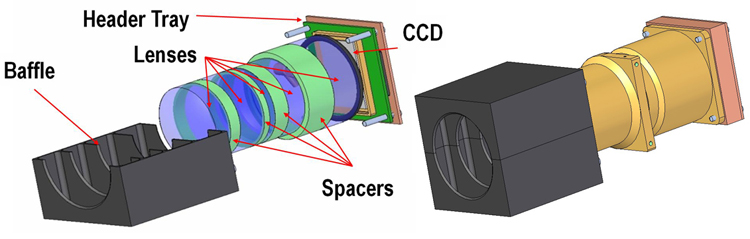
It is synonymously termed submillimeter radiation, terahertz waves, terahertz light, T-rays, T-waves, T-light, T-lux, THz. “ erahertz radiation refers to electromagnetic waves propagating at frequencies in the terahertz range. In terms of wavelength (λ), Planck's is written ī λ ( T ) = 2 h c 2 λ 5 1 e h c λ k B T − 1 Main articles: Radiation/Electromagnetics and Radiation astronomy/Electromagnetics
